How to make a fish face, and other photo contest winners
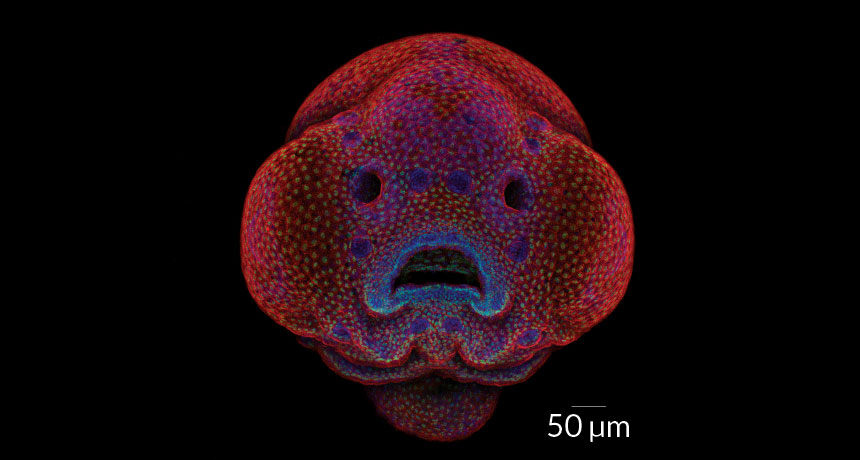
This forlorn-looking face of a 4-day-old zebrafish embryo represents “a whole new avenue of research” for geneticist Oscar Ruiz, who studies how faces and facial abnormalities develop at the cellular level.
The research is possible thanks to a new method, developed by Ruiz and colleagues at the University of Texas MD Anderson Cancer Center in Houston, for mounting embryos in a gel that allows for clear, head-on pictures. A technique called confocal microscopy captures images like the one above, the first-place winner of this year’s Nikon Small World photography contest.
The embryo was euthanized before having its picture taken. But Ruiz is experimenting with taking time-lapse images of live, anesthetized zebrafish embryos. The camera snaps an image every five minutes for up to 48 hours, meaning that “we’re able to watch the development happening,” Ruiz says.
So far, the team has looked at embryos ranging from 1 to 6 days old. The researchers are compiling the images into an atlas documenting the developmental stages of the zebrafish face. They plan to use CRISPR/Cas9, a powerful gene-editing tool, to alter genes involved in facial abnormalities in the fish and then watch what changes, if any, occur. The research could one day be used to help scientists understand how a cleft lip or cleft palate develops in humans and possibly help treat it, Ruiz says.
In the image above, shown at 10x magnification, basal cells (green) in the bottom layer of skin give rise to more developed surface skin cells (red). Cell nuclei appear in blue.
“Everyone’s first impression is that those two holes in the center are its developing eyes,” Ruiz says. But they’re not. Those deceptive hollows are nascent olfactory tissue, used to smell. The eyes are actually the big bulges on either side of the face.
Although this was the first time Ruiz entered the microscopy photography contest, he is an amateur photographer. Landscape or travel photos — not fish photos — are his subject matter of choice.
Here are more finalists and honorable mentions from the competition: